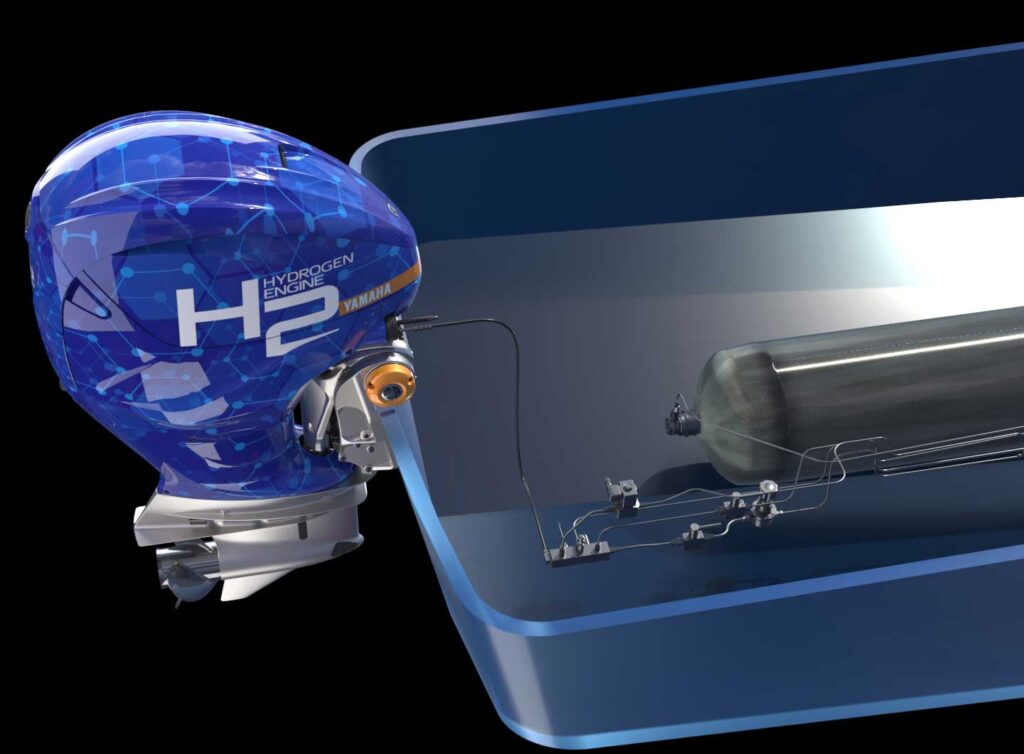
Yamaha Marine stole the limelight at the 2024 Miami International Boat Show in February when it revealed a 450 hp hydrogen-powered V-8 outboard. It featured a Roush Performance fuel-delivery system in a 26-foot 26 XO Regulator Marine hull. The deck was off to reveal three 6-foot-long cylindrical-shaped hydrogen fuel tanks and a custom stringer grid, to nest them securely and protect them from deformation in operation. At that time, preliminary testing of the motor and vessel indicated a range of 50 to 75 miles based on the estimated 23 kilograms of hydrogen pressurized to 10,000 psi in high-pressure, plastic-lined composite-overwrapped fuel tanks.
It brought up a big question: What does this portend for the future of recreational boating? Is this concept ready for prime time or just a pipe dream?
“It’s not optimized—yet,” says Grant Suzuki, chief technology engineer in charge of the marine innovation development division of Yamaha’s business unit.
Matt Van Benschoten, Roush vice president of advanced engineering, agrees. “A gas tank in that vessel would hold about 107 gallons of fuel,” he explains. “Our goal, for this first H2 fuel system, was to store approximately one-quarter of the gasoline energy content. The resulting fuel system achieves this goal.”
“The hydrogen initiative is an important pillar of three technologies on a pathway to carbon neutrality: hydrogen, electrification, and sustainable fuel,” Suzuki says.
Yamaha is not alone in its research. The National Marine Manufacturers Association has an alternative-fuel initiative that encourages and empowers manufacturers to develop lower-carbon fuels. Hydrogen is one of the NMMA’s favorites, but so are renewable fuels such as biobutanol, a distillate of organic materials.
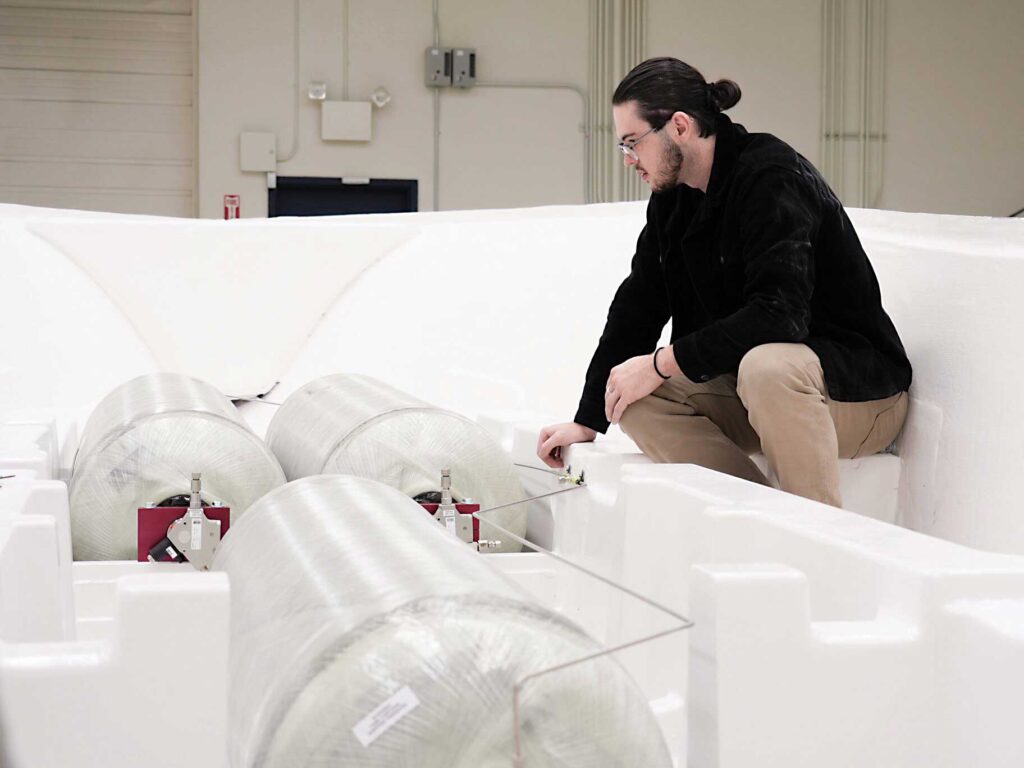
In the Beginning
In the 1980s, the California Air Resources Board began to demand lower emissions from fuel-thirsty two-stroke outboard engines that essentially drooled unburned fuel from their exhaust ports. By the late ’90s, Yamaha had perfected HPDI, regarded as the most reliable two-stroke direct-fuel-
injection system. It used computer wizardry to directly inject atomized fuel into the combustion chambers only after the piston stroke closed the exhaust port. Old two-stroke engines charged the combustion chambers while they were still open—a practice that was particularly wasteful at slower speeds. HPDI stopped the waste of blowing wet fuel through the inefficient two-stroke induction system, reduced emissions enormously, and maintained the power and torque for which two-stroke
tech was known. After that, in the early 2000s, Yamaha began research-and-development tasks using fuel cells and alternative fuels such as hydrogen.
At the same time, automakers such as Toyota, BMW and GM had well-developed fuel-cell prototypes. So did Yamaha in its motorcycle division.
Hydrogen fuel cells create electricity by initiating a chemical reaction between hydrogen and oxygen in a process called electrolysis. This electricity powers an electric motor—sometimes several, as in the case of numerous commuter buses operating in California. Several fuel-cell cars are on the market today, such as the Toyota Marai, but only 60 or so hydrogen fuel stations exist to refuel them. The cost most recently reported, in late 2023, was $36 per kilogram. In a fuel-cell Marai, that amounted to 50 cents per mile in fuel costs: green, maybe, but prohibitively expensive and not widely marketable. The exhaust produced by a hydrogen fuel cell consists of water vapor and warm air. Emissions are nil.
“That early work on fuel cells led us to experiment on the viability of hydrogen as an internal-combustion-engine fuel,” Suzuki explains of Yamaha’s efforts.
And that’s how the 26-foot Regulator concept boat with a hydrogen-powered Yamaha V-8 came to be and was placed on exhibit at one of the world’s most important boat shows.
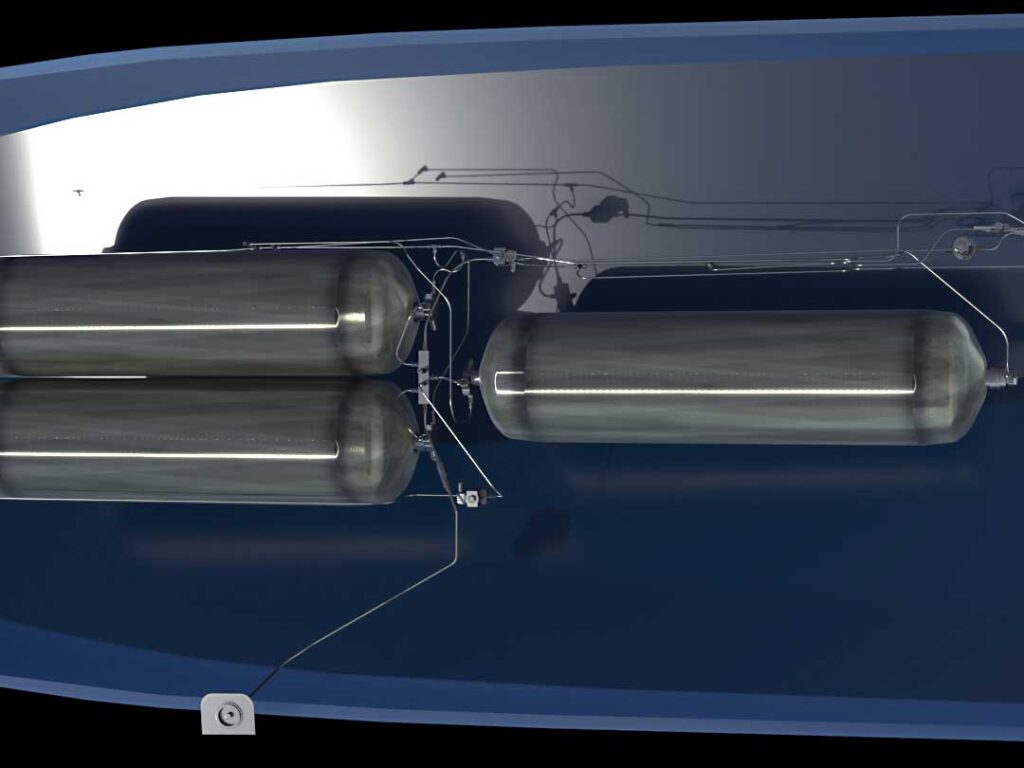
Hydrogen Pros and Cons
The potential advantage of hydrogen-fueled power lies in its use of conventional internal-combustion-engine technology, according to Yamaha. The twist is that the compressed hydrogen is delivered to the combustion chamber in a regulated manner, not atomized or vaporized gasoline forced in with the pressurized injection systems used in today’s engines.
Roush has decades of experience in this area, having developed many fuel-delivery systems for everything from aerospace applications to land-speed-record vehicles. Roush’s systems replace conventional port or direct-injection systems and engine control modules, and often integrate supercharging technology.
Roush’s Van Benschoten explained the challenges of the fuel-delivery system. “Three Type 4 tanks hold about 7.5 kilograms of hydrogen [per tank] at 700 bar (10,000 psi),” he says. “Type 4 tanks are made with a plastic liner and composite overwrap. Tanks expand and shrink during each fuel-burn-refuel cycle, so it’s important to design a mounting system that does not overconstrain the tanks.”
Clearly the engine and fuel system are a heavy lift, but according to Joan Maxwell, Regulator’s president and CEO, her company is up to the task.
“One of the challenges of this project was simply trying to place highly pressurized tanks in existing hulls,” she says. “For us at Regulator, it was a proof of concept.”
Regulator enlisted its top engineers to reform the 26 XO’s stringer grid to accept the tanks and protect them from deformation during use and abuse, then fit that into a boat. The design had to accommodate high-pressure fuel lines to transfer hydrogen to the fuel-delivery system at a rate to let the engine develop optimal horsepower, as well as accommodate the tanks’ expansion and contraction during burn and refuel cycles.
From 700 bar in the tanks, the hydrogen pressure must be stepped down through a series of regulators to 100 bar, or about 1,500 psi, which is the pressure at which the fuel injectors deliver the H2 into the combustion chambers.
Engineering challenges of fuel delivery include managing the ratio of oxygen to hydrogen being delivered into the combustion chamber and controlling ignition timing appropriately to account for the shorter burn duration of hydrogen-air versus gasoline-air vapor. The process, naturally, requires custom computerized engine controls.
“Another challenge is the potential embrittlement of steel alloys, which, when not selected appropriately for a hydrogen-rich environment, can experience a reduction in the ductility due to absorbed hydrogen,” Van Benschoten says.
Hydrogen will diffuse into metals, and even after the fuel has left the system, it remains in the metal, and over time, that can cause it to fracture prematurely.
“We have to design around that, particularly in the fuel-delivery system,” he says. “It also impacts the cylinders and heads and pistons, but the most vulnerable parts are the injectors and fuel rails and lines. You’ll see a lot of stainless steel in those parts.”
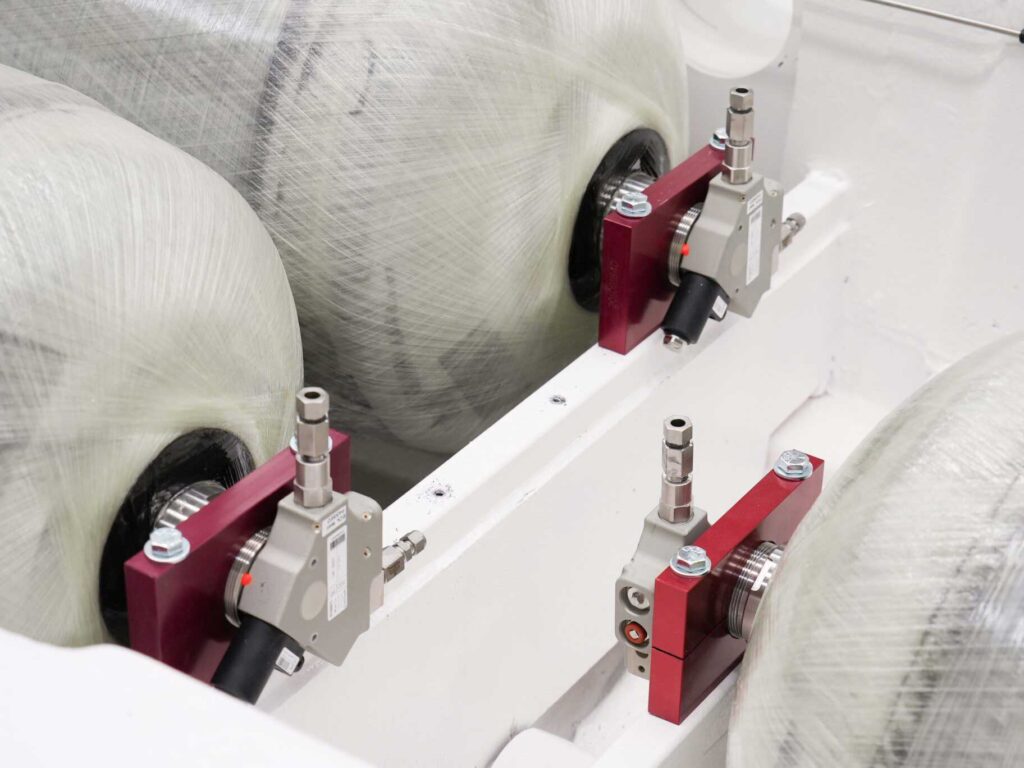
Combustible Confusion
You can’t discuss hydrogen as a fuel without thinking of the hydrogen-filled Hindenburg, which burst into flames when the German lighter-than-air passenger-carrying airship ignited while attempting to touch down in Lakehurst, New Jersey, in 1937. The disaster killed 36 people and injured more than 50 others. Anecdotally, recent studies have offered convincing evidence that it was the highly flammable doping compound painted on the fabric of the dirigible that was ignited by a spark, not the hydrogen inside. Still, many understandably worry that hydrogen fuel is dangerous because it’s extreme volatility—or so the thought goes. However, gasoline fumes are comparably volatile and are heavier than air, so if a gas tank ruptures and the fuel escapes initial ignition, the fuel is still present, dripping into a boat or floating on the water, at continued risk of ignition. Hydrogen will be evacuated from a compromised fuel tank almost immediately, provided that there is a way for it to get out of the boat, thus minimizing the time in which combustion can occur.
Van Benschoten explained Yamaha’s safety enhancements for handling hydrogen on board: “We have ‘sniffers’ in the bilge to detect hydrogen leaks, and if there is one, the tank valves are automatically closed, isolating the tanks, and the fuel lines are purged and piped above the top of the vessel, where it continues to rise and dissipate in the air. The process takes about 15 seconds. We also have vents in the bilge, but their pickups are on the highest points in the bilge—not the lowest, as they would be with gasoline, since hydrogen is lighter than air.”
So, place your bets: Which is safest? Gasoline? Hydrogen? Let’s not even talk about the potential hazards of lithium batteries.
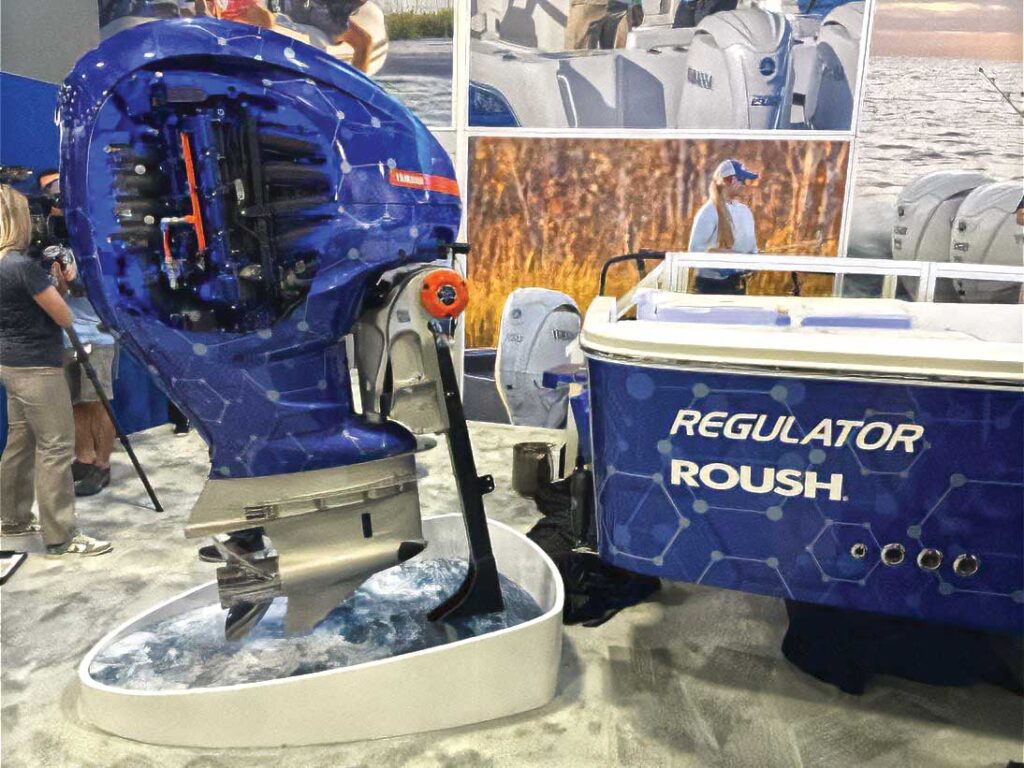
Where to Source Hydrogen
Hydrogen is an element, the first and simplest one on the periodic table, with only one proton and one orbiting electron. It takes two hydrogen molecules bound together to make the hydrogen gas that burns—H2 is its chemical designation. Hydrogen makes up 75 percent of everything—me, you, the stars, the sun—and ranks as the most abundant element in the universe. To extract hydrogen gas, it must be separated from water (H20), natural gas or other fossil fuels such as coal. The processes, and the carbon footprints of each, are identified by color. Green hydrogen is separated from water using renewable (solar or wind) electricity to power the electrolysis process and costs the most to produce. It is scarce. Blue H2 is produced from fossil-fuel-powered electrolysis, but carbon dioxide is captured and repurposed or stored. Gray hydrogen is the most plentiful and is separated from natural gas. Brown or black hydrogen is the easiest, cheapest and dirtiest to make, from coal in a gasification process. The cost to produce hydrogen ranges from $1 per kilogram to $6 per kilogram. Unfortunately, due to distribution costs and poorly developed infrastructure, the price at the pump is an astronomical $36 or more per kilogram. Moreover, all existing and potentially known sources of hydrogen fall short of the current and projected future needs for world transportation.
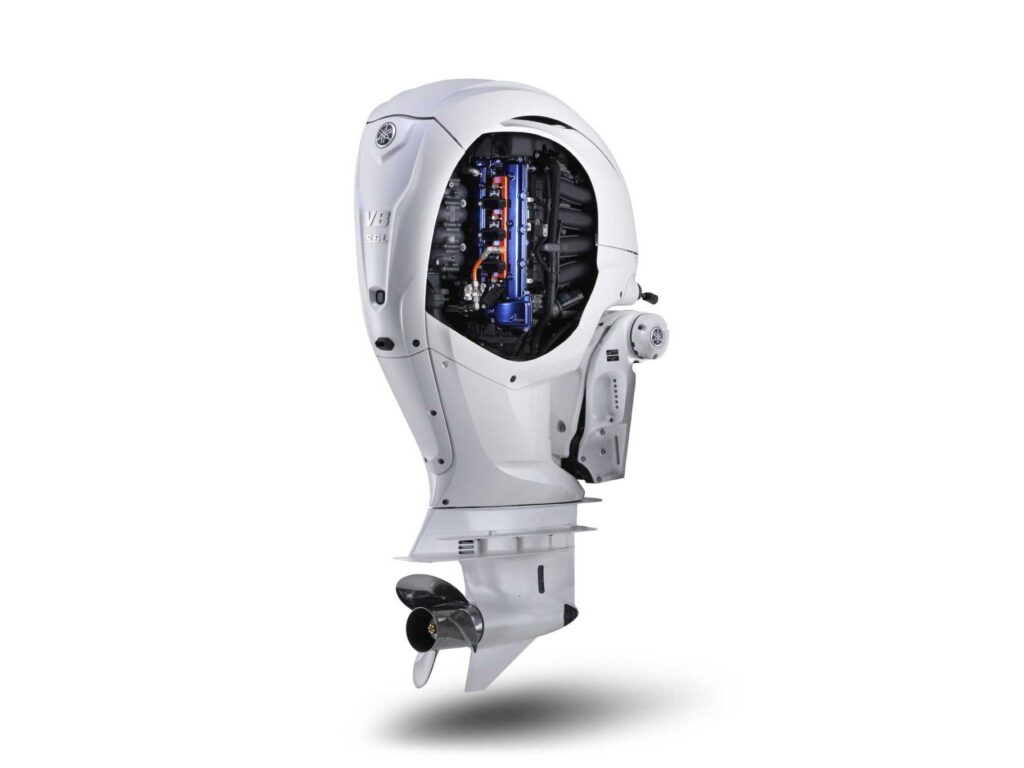
Getting Hydrogen in the Tank
Regardless of where hydrogen is sourced, it faces its next big and costly challenge: getting it to users. It can be transported by pipeline, and often is for large users such as gasoline refineries.
It can be transported as compressed gas, but that’s far more costly for the amount of fuel carried in a tanker truck as compared with gasoline or diesel. It can be transported liquefied more easily, but its propensity for shrinkage is high, and the energy required to compress it, plus complications and liabilities at the point of delivery, adds to that cost.
Transporting H2 is an energy-burning process too. The gasoline refining industry limits hydrogentransport costs frequently by locating near hydrogen-producing plants. That eliminates transportation as one of the big energy consumers for them. At some point, hydrogen-production plants could be scalable to locate at or near fuel service stations. But at present, door-to-door hydrogen delivery is not on the horizon, and at this writing, there are fewer than 100 hydrogen fuel stations in North America. Most are in California. Broad distribution of it is not practical. Yet.
Read Next: Decarbonization of Boating
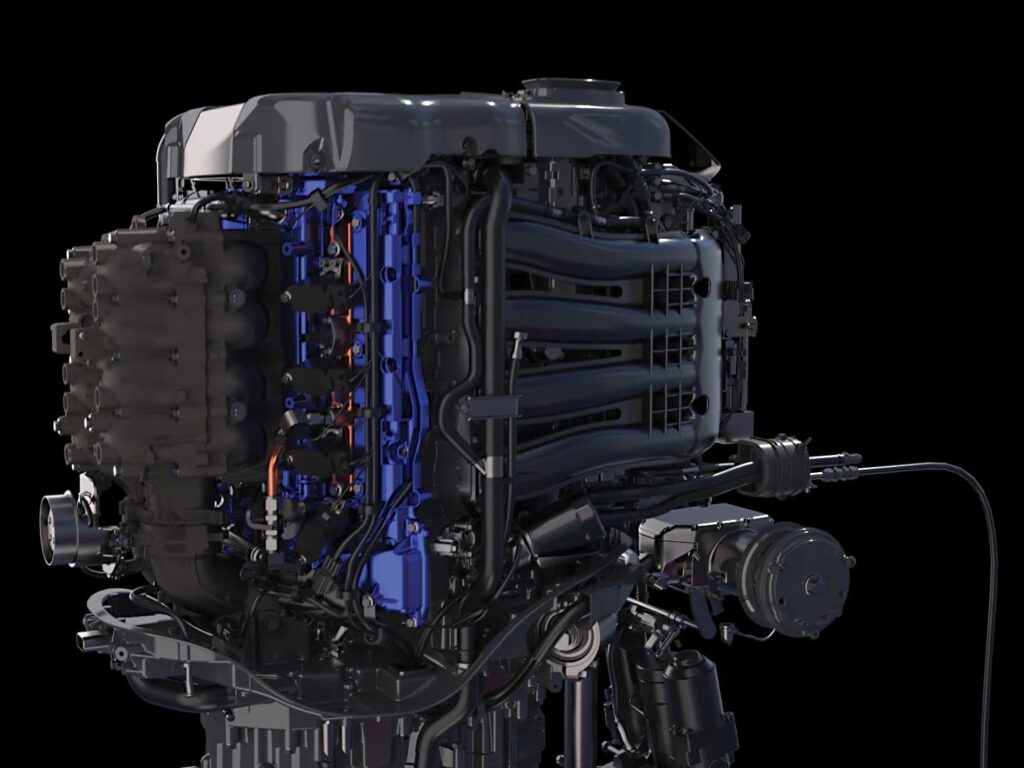
If Not Now, When?
So, if Yamaha perfects its hydrogen engine in, say, two years, would we see them on the water in growing numbers? Probably not. Look back to the early 2000s, when carmakers such as Toyota, Honda, BMW and GM, as well as motorcycle-makers such as Yamaha, had extensive experience developing fuel cells, but the Western world began to push electric propulsion because transportation of carbon-neutral fuel—mainly hydrogen—was a long way from addressing the ubiquitous distribution system enjoyed by gasoline or even the electric power grid. Now, however, as that demand for electricity increases, the need for an alternative green fuel increases, which ultimately might generate a deeper dive into hydrogen power.
Should Boaters Care?
If Roush’s estimates are correct, 23 kg of hydrogen can provide energy comparable to 26 gallons of gasoline. At the current street price of $36 per kilogram, a 23-kilogram hydrogen fill-up would cost $828 (at $36 per kilogram) and propel the boat about 50 miles, while a 107-gallon gas fill-up would be $642 at the waterfront and get the boat about 180 miles. And, even when highly compressed, hydrogen takes up almost four times the space of gasoline for the equivalent amount of energy.
As with electric propulsion, hydrogen is not the formula for every challenge. And H2 is far from viable today. But, as Yamaha, Roush and Regulator see it, it’s one potential heading in the passage to a carbon-neutral world.